Mechanisms of
|
2019 Video Challenge
Winners | Entries | Judges | Overview | Learning Resources
Note: Videos are intended for communication and not rigorous scientific review.
Congratulations to the 2019 Winners:
Judges' Award First Place
The Criminal Case of the Aminoglycoside Misuser
By Brean Bognot and Cayla Tolentino of Mira Mesa High School, San Diego, CA
Team Advisor: Mrs. Lisa Yoneda
Judges' Award Second Place
The New Arms Race: Aminoglycoside Antibiotics
By Anvi Surapaneni and Vivian Hir of The Quarry Lane School, Dublin, CA
Team Advisor: Mrs. Alina Hamm
Judges' Award Third Place
The Three Little Bacteria and the Big Bad Tobramycin
By Carlos Hernandez, Jeff Huang, and Shamir Sheikh
of Stuyvesant High School, New York, NY
Team Advisor: Mr. Gilbert Papagayo
Viewers' Choice Award
Mechanisms of Aminoglycoside Antibiotic Resistance
By Charumathi Badrinath
of Rye Country Day School, Rye, NY
Team Advisor: Mrs. Jennifer Doran
2019 Challenge Details
In this challenge, students were asked to tell a story that communicating 2 things:
-
The molecular changes that occur in bacteria that help them to become resistant to aminoglycoside antibiotics using relevant 3D protein structures.
Videos were required to include a general introduction the biological function of ribosomes, a short description of how antibiotics affect the ribosomes, and a segment devoted to one of the aspects of the bacterial resistance:
- Inactivation of the antibiotics via aminoglycoside modifying enzymes
- Modifications of ribosomes by methyltransferases
- Extraction of antibiotics from the bacterial cells via multidrug resistant efflux pumps
The dangerously high level of antibiotic resistance caused by misuse and overuse of antibiotics. Students were required to explain how viewers might be affected, and what they can do prevent it.
2019 Dates
The video submission opened on January 15, 2019 and concluded on April 23, 2019. Award winners were announced at rcsb.org on May 14, 2019.
The section below was avialable to the participants as introduction to the topic, and offred links to relevant 3D structures and additional resources.
2019 Learning Resources
I. What are antibiotics?
Antibiotics are small molecules, originally discovered as defensive molecules made by fungi and other organisms, and now created by medical scientists. They bind to essential bacterial molecules, such as enzymes and ribosomes, blocking the growth of the bacteria or killing them. Because these bacterial proteins are structurally different from their animal equivalents or are non-existent in them, they cause no harm to the organism treated for bacterial infection.
Figure 1 presents main classes of antibiotics and their target protein/molecule organized by the biochemical process that they interrupt in bacteria. In this video challenge, we will be focusing only on the action of and resistance to the aminoglycoside class.
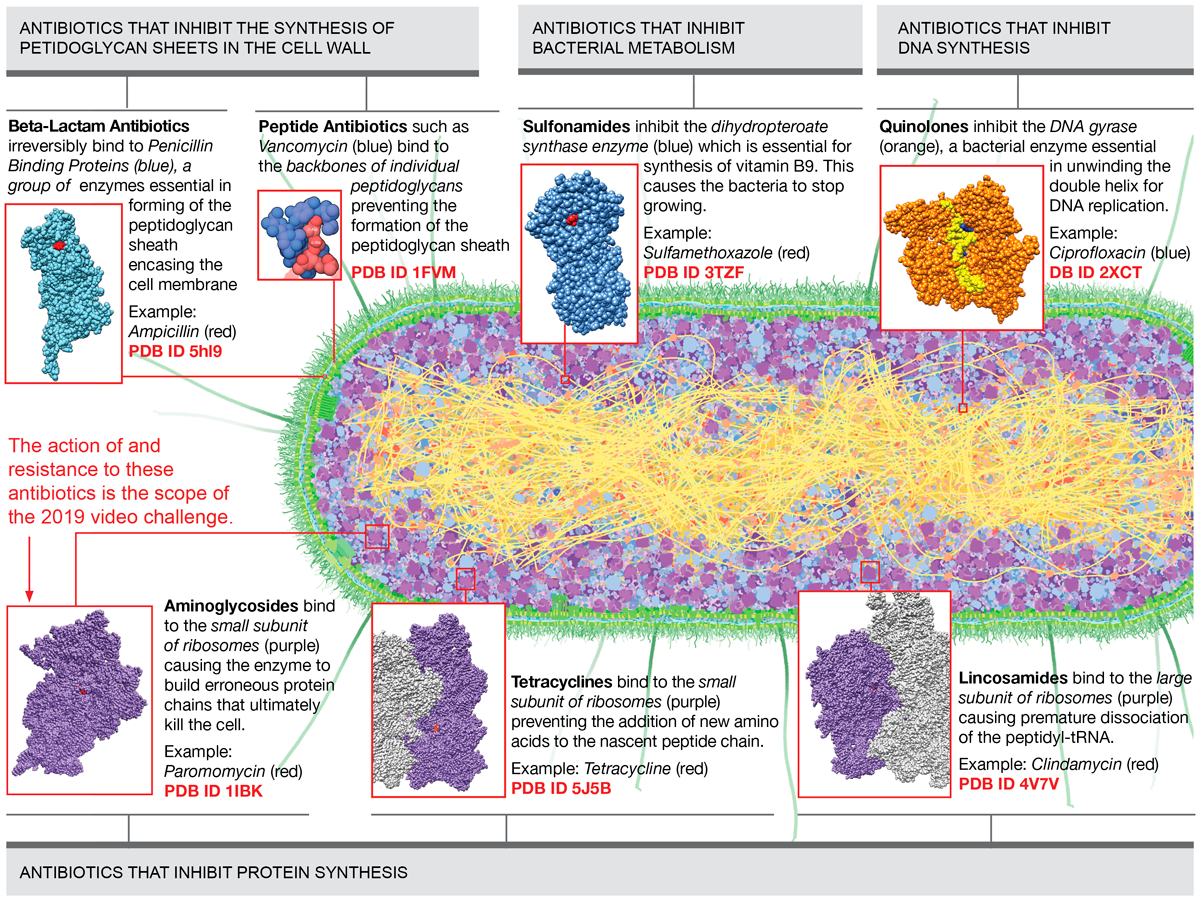
Figure 1
Main classes of antibiotics and their target protein/molecule organized by the biochemical process that they interrupt in bacteria. Download as PDF
II. How aminoglycoside antibiotics work
The aminoglycoside antibiotics are made by some bacteria to protect themselves from competing bacteria. They are particularly effective because they are very specific: they attack bacterial ribosomes, corrupting protein synthesis in the bacterium, but they don't attack the ribosomes of many other organisms, including our own ribosomes.
Ribosomes are the molecular machines that build new proteins based on the genetic information carried in the messenger RNA. Ribosomes are made up of multiple protein chains and rRNA that form the small and the large subunits. To learn more about the ribosomal subunits, read the Molecule of the Month on the topic.
Figure 2 shows eukaryotic and bacterial ribosomes drawn to scale and highlights the key differences in the nomenclature of their key components.
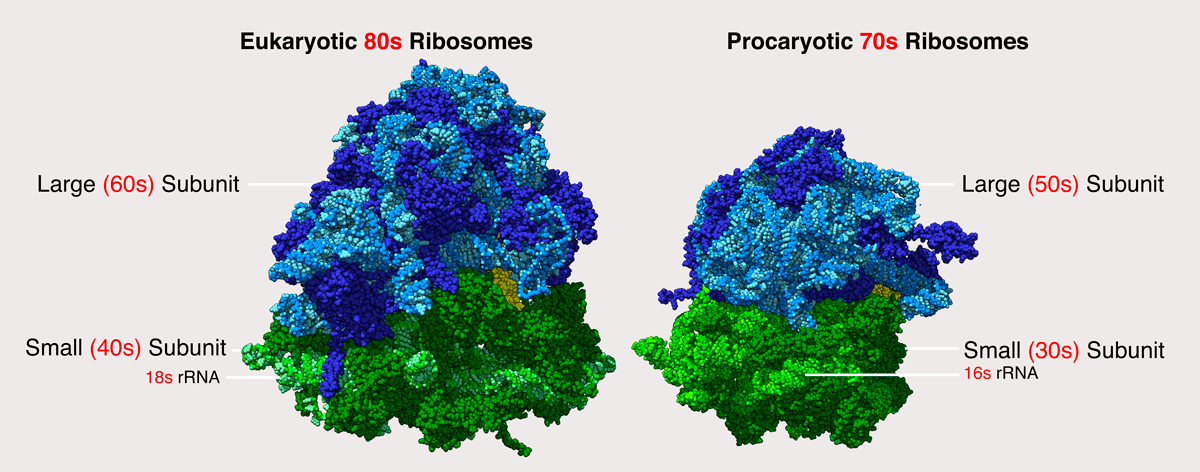
Figure 2
Eukaryotic ribosomes; PDB ID 6ek0 and prokaryotic ribosomes; PDB ID 4v5d.
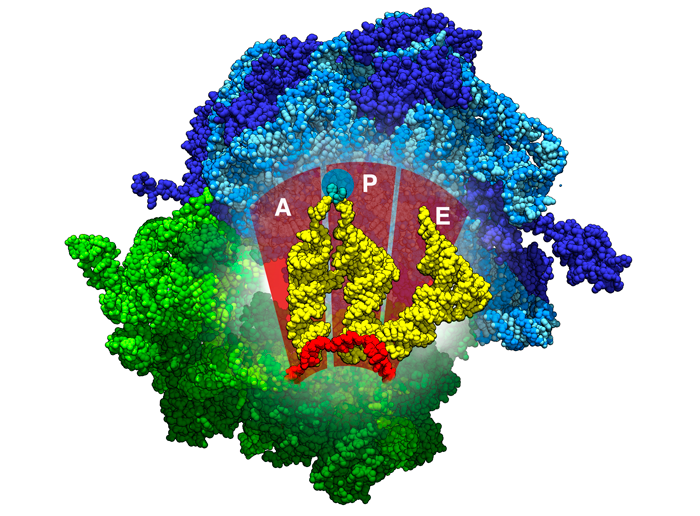
Figure 3
Location of the A, P, and E binding sites inside ribosomes. tRNAs are shown in yellow, the mRNA in red, the beginnings of the polypeptide chain in aqua. PDB ID 4v5d
Despite structural differences, the ribosome in bacteria and prokaryotes follow the same steps in protein synthesis. Once the messenger RNA and a special initiator tRNA (different for prokaryotes and eukaryotes) bind to the smaller subunit of ribosomes, both subunits come together, creating the enzymatic environment for creating new protein chains. The stages of protein synthesis are described in the Molecule of the Month on Ribosome.
The ribosomes have 3 binding sites for the tRNA: A (acceptor site), P (peptidyl site), and E (exit site). Figure 3 shows the locations of these sites inside the ribosomes. The A (acceptor site) site is where the a tRNA carrying a matching codon to mRNA binds. During this step, the ribosome uses several interactions to test this pairing, ensuring that the base pairing is correct. You can explore these interactions in 3D in the Exploring the Structure section, in the Molecule of the Month on Ribosome.
The aminoglycoside antibiotics bind at the A site and they interfere with the recognition of the the matching tRNA, ultimately leading to mistakes in building the new protein chains. You can explore the aminoglycoside binding site in 3D in the Exploring the Structure section of the Molecule of the Month on Aminoglycoside Antibiotics.
Another mode of action for the aminoglycoside antibiotics is binding to the large subunit of the ribosome and causing problems at the end of protein synthesis, blocking the recycling of ribosomes after they are finished making a protein. You can explore this process in the Structural Biology Highlights article Antibiotics and Ribosome Function.
Aminoglycoside antibiotics are used mainly to treat infections caused by gram-negative bacteria in a clinical setting.
Table 1: Examples of Aminoglycoside Antibiotics and PDB structures with the antibiotic bound
Antibiotics and core structure | Description | Example PDB structure with the antibiotic bound | Visualization resources and tips | Learning Resources |
![]() |
|
1fjg
|
This structure shows the small subunit of the ribosomes (colored green in the Chimera session) with streptomycin (SRY - red) attached in the active site. Chimera session (preview below)b ![]() |
Molecule of the Month on Aminoglycoside Antibiotics Featured System article Antibiotics and ribosome function 2018 calendar Mechanisms of Antimicrobial Resistance page for May
|
![]() |
|
4v53
|
This structure shows the large and small ribosomal subunits (colored blue and green respectively in the Chimera session) with gentamycin (LLL - red) attached in the small subunit’s active site (interfering with the protein production) and in the large subunit’s active site (blocking the recycling of the ribosomes). Chimera session (preview below)b ![]() | |
![]() |
|
4lfc
|
This structure shows the small subunit of the ribosomes (colored green in the Chimera session) with tobramycin (TOY - red) attached in the active site. Chimera session (preview below)b ![]() |
III. Mechanisms of bacterial resistance to aminoglycoside antibiotics
In the face of overuse and misuse of antibiotics, new strains of bacteria have emerged that can proliferate even in the presence of the newest antibiotics. There are multiple ways the bacteria adapt to develop resistance against the aminoglycoside antibiotics. Of current clinical prominence are the following three molecular mechanisms:
- Aminoglycoside modifying enzymes
- Ribosomal methyltransferases
- Efflux pumps that remove the antibiotics from the bacterial cell
There are two ways in which the resistance genes for each of these mechanisms can be passed on: via Chromosome, where the gene is transferred from generation to generation (vertical transfer) and via a plasmid that can be passed from bacterial species to species (horizontal transfer).
1. Aminoglycoside modifying enzymes
Aminoglycoside modifying enzymes alter the structure of antibiotics directly so that they can’t attach to their target. They are a large family containing three subclasses based on the type of modification that they apply to the the antibiotic:
O-nucleotidyltransferase (ANT):
these enzymes add a nucleotide to the drug
Before Reaction: Tobramycin: TOY | After Reaction: Adenylated Tobramycin 51H |
|
![]() |
---------> |
![]() |
N-acetyltransferases (AAC):
these enzymes add an acetyl group to the antibiotic
Before Reaction: Gentamycin: LLL |
After Reaction Acetylated Gentamicin 8MM |
|
![]() |
------> |
![]() |
O-phosphotransferase (APH):
these enzymes connect a phosphate to the drug
![]() |
Table 2: Example PDB entries for aminoglycoside modifying enzymes
Example PDB structure |
Expressing Gene |
Visualization hints and resources |
Learning resources |
O-nucleotydyltransferase (ANT): Before Reaction: After Reaction: |
aadB |
PDB entries 5cfs and 5cfu (colored blue in the Chimera session) show the enzyme before and after the reaction. Tobramycin is orange. In the “before” structure, a non-reactive analog of ATP (yellow) is used to freeze the reaction, and in the second structure, the nucleotide (colored lighter orange) has been attached to the antibiotic. Chimera session (preview below)b ![]() |
Molecule of the Month on Aminoglycoside Antibiotics and Resistance Molecule of the Month on Aminoglycoside Antibiotics 2018 calendar Mechanisms of Antimicrobial Resistance page for June Superbugs! How Bacteria Evolve Resistance to Antibiotics Poster |
N-acetyltransferases (AAC): 1bo4 |
aacC1 |
The structure contains 2 subunits (colored shades of blue in the Chimera session). Each subunit has coenzyme A (COA - yellow) bound in the active site. Spermidine (SPD - orange) is bound in the gentamicin-binding site. Chimera session (preview below)b ![]()
|
|
O-phosphotransferase Crystal Structure Of 3',5"-Aminoglycoside Phosphotransferase Type IIIa ADP Kanamycin A Complex |
aphA |
Aminoglycoside Phosphotransferase (colored blue in the Chimera session) is bound to Kanamycin (KAN – Orange) and ADP (yellow). The phosphate group colored darker yellow will be attached to Kanamycin during the reaction. Chimera session (preview below)b ![]() |
2. Ribosomal methyltransferases
The ribosomal methyltransferases modify ribosomes enzymatically by adding methyl groups to a nucleotide on the 16S rRNA in the aminoglycoside binding site. This does not affect the function of the ribosome, but prevents the antibiotic from binding.
Table 3: Example PDB structures for ribosomal methytransferases
Example PDB structure |
Expressing Gene |
Visualization hints and resources |
Learning resources |
4ox9 |
npmA |
The structure shows small ribosomal subunit (colored green in the Chimera session) with the methyltransferase enzyme (Chain Y - magenta) bound to it. The key nucleotide (Adenine 1408) that the enzyme will add methyl group to in the course of the reaction is highlighted in lighter green. Chimera session (preview below)b ![]() 4ox9 NGL view a |
Molecule of the Month on Aminoglycoside Antibiotics and Resistance Molecule of the Month on Aminoglycoside Antibiotics Superbugs! How Bacteria Evolve Resistance to Antibiotics Poster |
3frh |
rmtB |
The structure shows the methyltransferase enzyme (colored magenta in the Chimera session) with S-adenosyl-L-homocysteine (SAH - green) bound in the active site. The S-adenosyl-L-homocysteine has a great structural similarity to S-Adenosyl methionine, the molecule that the enzyme normally uses to extract the methyl group from and add it to the nucleotide on the rRNA. Chimera session (preview below)b ![]() |
3. Efflux pumps that remove the antibiotics from the bacterial cell
Multidrug resistance transporters or efflux pumps find drugs that try to gain entry through a cell membrane and they transport them back outside.
Bacteria possess multidrug resistance (MDR) gene regulators that can sense when the antibiotics get into the bacterial cell, and prompt the synthesis of the multidrug resistance pumps to eject them.
Table 4: Example of efflux pump and a multidrug resistance (MDR) gene regulator
Example PDB structure |
Expressing Gene |
Visualization hints and resources |
Learning resources |
Gram-negative bacteria multidrug efflux pump: 5o66 |
tolC, acrA, acrB, acrZ |
The structure shows an efflux pump from Gram-negative bacteria. For your reference, the inner and outer membranes are highlighted on the Chimera session preview. Chimera session (preview below)b ![]() |
Molecule of the Month on Multidrug Resistance Transporters 2018 calendar "Mechanisms of Antimicrobial Resistance" pages for November and December Superbugs! How Bacteria Evolve Resistance to Antibiotics Poster |
Multidrug resistance (MDR) gene regulator 3q3d |
bmrR |
The structure shows a fragment of bacterial DNA (colored yellow on the Chimera session) with the gene regulator bound to it. The gene regulator has puromycin (orange) bound in each subunit. Chimera session (preview below)b ![]() |
Visualization Resources
- The user guide for NGL can be found here. The NGL is the default 3D viewer accessible from each structure summary page, from the tab “3D View”.
- You can download and open these sessions using UCSF Chimera.
Use the tutorials available here and here to edit the sessions, create animations or save pictures.