2021 Video Challenge for High School StudentsMolecular Mechanisms
|
2021 Video Challenge
Winners | Entries | Judges | Overview | Learning Resources
Note: Videos are intended for communication and not rigorous scientific review.
Congratulations to the 2021 Winners:
Summary of 2021 Video Challenge
The 2021 Challenge Introduction
Mental disorders affect thinking, feeling, mood, and behavior. As scientists study the molecular machinery behind brain processes and mental illnesses, they design drugs that bind to key proteins in the neuronal signaling pathways to alter their innate function. This in turn regulates the neuronal signal strength. Although these drugs don’t cure mental disorders, they help patients lead more productive and fulfilling lives.
In this challenge you will focus on a mental health topic and create a story that explains how neuronal signals are communicated within our bodies and how pharmacological drugs modulate that signal in a given pathway to treat the symptoms of the illness. Choose one of the topics below:
-
Treating anxiety disorders: drugs targeting GABAA receptor to reduce neuronal stimulation
-
Treating schizophrenia: pharmacological antagonists targeting the dopamine receptor
-
Treating depression: drugs targeting SERT transporters to modulate the reuptake of serotonin
-
Treating Alzheimer’s disease: drugs targeting acetylcholinesterase to stop the destruction of acetylcholine
In addition, your story should incorporate some social and public health aspects. You might address how the mental illness affects an individual and how that could be improved with increased awareness. You might also convey the importance of screening for the disorder.
2021 Challenge Dates
Submission | January 12 - April 27, 2021 |
Judging | May 3 - May 10, 2021 |
Results Announced | May 18, 2021 |
2021 Learning Resources
- Introduction
- Signaling with Action Potentials: transmitting the signal along a neuron
- Signaling with Synaptic Transmission: passing the signal from neuron to neuron
I. Introduction
Our nervous system regulates myriad of tasks in our organisms, from muscle movement and blood vessel dilation to thinking, learning, and our emotions. Each task is assigned to a circuit of neuronal cells which receive and dispatch the signals. Despite the complexity of the tasks and abundance of the neuronal pathways, the underlying molecular machinery is fairly uniform throughout the whole system. The signal within the cell is carried as action potential–a positive charge traveling along the neuron. The signal is then passed onto another neuron via release of small chemicals called neurotransmitters into intraneuronal space. These small molecules stimulate neighboring cells by binding to appropriate receptors. Once the message is passed, the neurotransmitters have to be quickly removed from the space between neurons to stop stimulating the target cells. Some types are transported back into the cell, some of them are destroyed by special enzymes.
Learn More:
Learn about the structure of the nervous system from Khan Academy
II. Signaling with Action Potentials
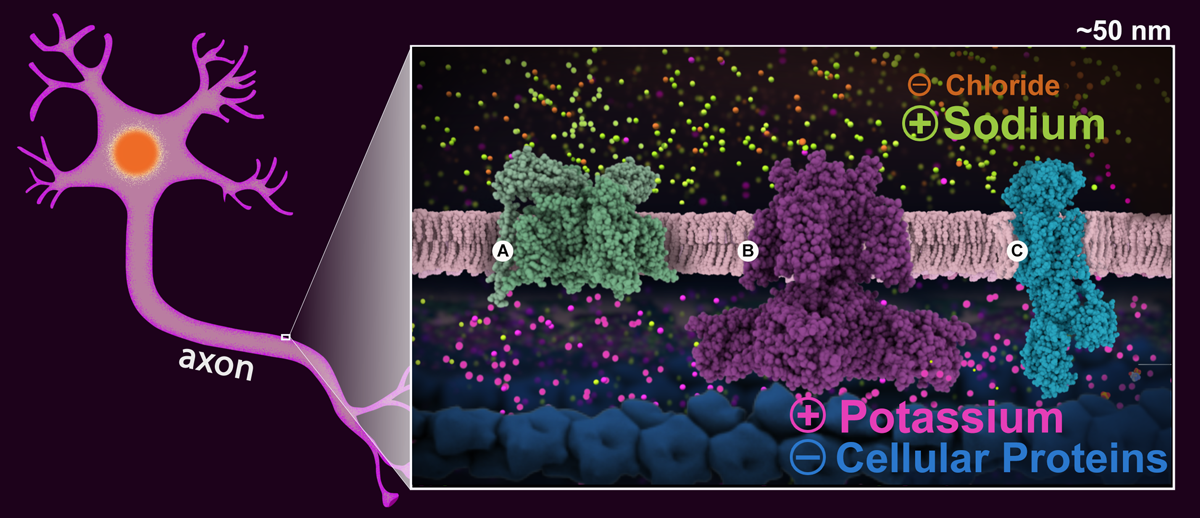
Figure1. Schematic drawing of a neuronal cell with a ~50 nm section of the axon highlighted. The enlargement shows the cell in the resting state highlighting the sodium (green) and potassium (magenta) ion gradients, and the positive and negative charges distribution on both sides of the neuronal membrane. Proteins essential for signal transmission are shown: A: voltage gated sodium channel (PDB structure 5x0m), B: voltage gated potassium channel (PDB structure 6pbx), and C: sodium-potassium pump (PDB structure 2zxe).
Positively-charged sodium and potassium ions are the signaling agents that carry signals down the long axons of nerve cells. This happens in several steps. First, the sodium-potassium pump (Figure 1C) transfers sodium outside the cells, and potassium inside the cell. This along with other molecules and atoms creates a voltage gradient across the cell membrane (of -70 mV), which is used to power the fast transmission of the nerve signal. This state is refered to as "resting potential" and the membrane is said to be polarized (Figure1).
When the cell is triggered to send a signal, voltage-gated sodium channels (Figure 1A) open in the area near the start of the axon. This allows sodium ions to enter the cells, changing the voltage across the membrane to about +40 mV. This triggers more channels further along the axon to open, changing the voltage there, depolarizing the membrane. This wave of channel opening, termed the "action potential", sweeps down the axon.
Soon after, voltage-gated potassium channels (Figure 1C) in the area also open, allowing potassium ions to leave the cell, stopping the action potential. Once the voltage inside reaches about -75mv they close and the membrane is said to hyperpolarized. During this brief period another action potential cannot be triggered.
The membrane is restored to its -70mV resting potential value thanks to special potassium channels that allow the potassium to travel back inside the cell. Finally, the sodium-potassium pump begins transferring the sodium back out and the potassium back in, making the axon ready for another signal.
Learn more:
Molecule of the Month articles:
Voltage-gated Sodium Channel
Potassium Channel
Sodium-Potassium Pump
III. Signaling with Synaptic Transmission
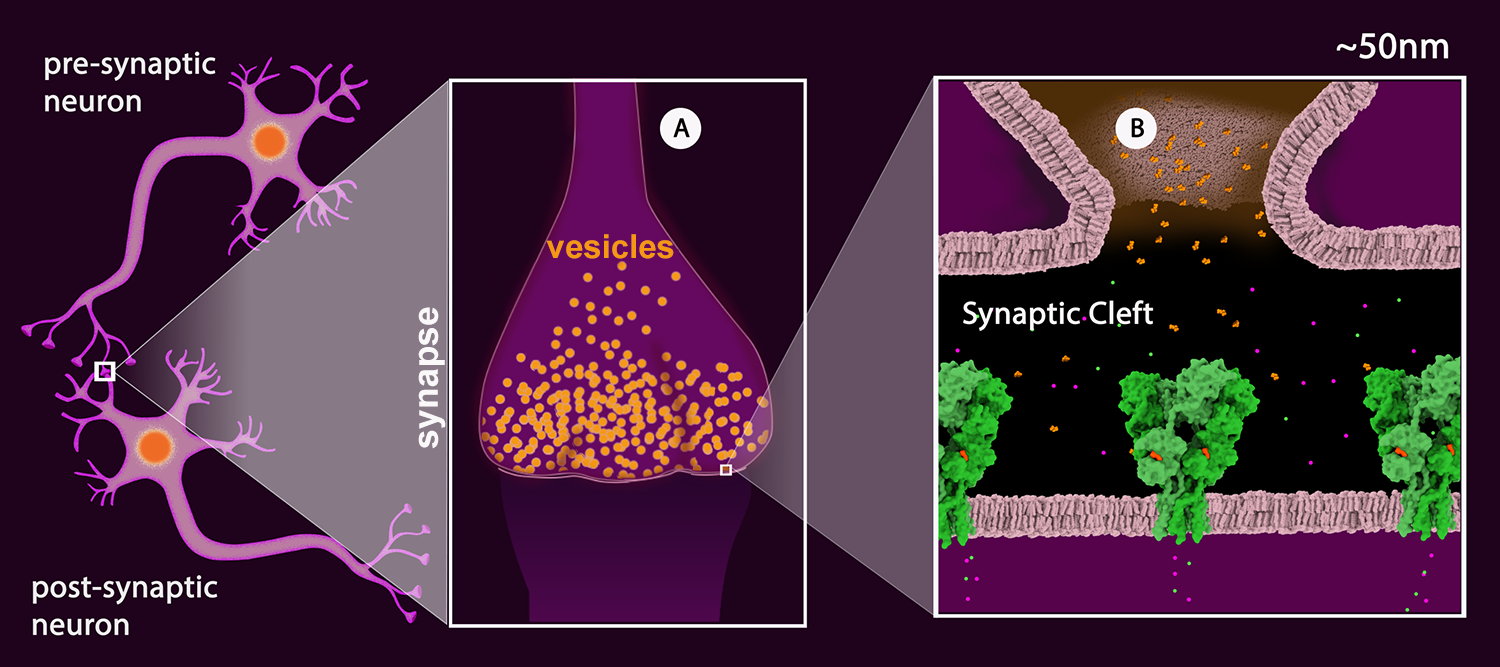
Figure 2: Schematic drawing of a neuronal junction with the synapse highlighted. A: Synaptic vesicles (orange). B: View of the synaptic cleft showing one vesicle merged with the pre-synaptic membrane and the neurotransmitter (glutamate - orange) released into the cleft. The neurotransmitter receptors are shown in green. (AMPA Receptor, PDB Structure 3kg2). The receptor is a ligand-gated ion channel permeable to sodium (green) and potassium (magenta).
1. Neurotransmitters
Once the action potential reaches the terminal region of the neuron, called the synapse (Figure 2A), the signal is passed onto the next neuron (termed the “postsynaptic neuron”) by the release of neurotransmitters. These chemical messengers are small molecules or short peptides, stored in synaptic vesicles. When the action potential arrives, these vesicles fuse with the neuronal membrane, releasing neurotransmitters into the synaptic cleft. Once released, the neurotransmitters bind to matching receptors on the postsynaptic membrane (Figure 2B). There are many different neurotransmitters used for different jobs: glutamate excites nerves into action, GABA inhibits the passing of information, dopamine and serotonin are involved in the subtle messages of thought and cognition, and acetylcholine carries signals from nerve cells to muscle cells.
![]() |
![]() | ![]() |
![]() |
![]() |
Learn more:
SciArt Gallery:
Excitatory and Inhibitory Synapses
2. Types of neurotransmitter receptors
Neuroransmitter receptors span the membranes of post-synapic neurons. The receptors can be classified into two broad categories: metabotropic and ionotropic. This classification is based on their structural and functional characteristics.
2a. Ionotropic Receptors
The ionotropic receptors, also called ligand-gated ion channels, contain an ion channel that opens upon neurotransmitter binding allowing the ions to flow through the channel. This event is very short and ions only flow for as long as the neurotransmitter is bound to the receptor. Once the neurotransmitter detaches, the receptor goes back to its closed confirmation. Depending on the type of neurotransmitter used and the receptor, they create inhibitory or excitatory postsynaptic potentials.
For example, an excitatory postsynaptic potential is created through glutamate interacting with AMPA receptor. Upon glutamate binding, the receptor channel opens to allow entry of positively-charged sodium, potassium, and calcium ions. The incoming ions depolarize the membrane, generating an action potential that travels down the neuron.
Inhibitory postsynaptic potential is created, for example, when GABA interacts with the GABAA receptor. Upon GABA binding, the receptor allows the influx of negatively charged chloride, which hyperpolarizes the membrane, making it more difficult to start a new action potential.
Some neurons in our nervous system have synapses with both neurons sending excitatory signals and neurons sending inhibitory signals. The positive charge entering the neuron from the excitatory signal can be offset by the negative charge from the inhibitory neuron signal. In such cases, no action potential can be generated and the signal is halted. In this way, the neuron integrates the different inputs from many neurons, only sending a new signal when enough excitatory signals are received.
Learn more:
Molecule of the Month articles:
Acetylcholine Receptor
AMPA Receptor
Glutamate-gated Chloride Receptors
SciArt Gallery:
Excitatory and Inhibitory Synapses
Pharmacological application: Targeting GABAA receptor in treating anxiety disorders
The GABAergic neurons (neurons using GABA as primary neurotransmitter) are associated with the modulation of anxiety responses. A group of drugs prescribed to treat anxiety disorders called benzodiazepines (e.g. Valium) stimulate the GABAA receptor. Binding of the drug to an allosteric site on the receptor prolongs the binding of GABA, which in turn increases the amount of chloride ions entering the neuron resulting in hyperpolarization.
PDB Structure ID |
Structure Description |
Visualization resources |
Topic-specific resources |
3D structure of GABAA receptor in complex with GABA and diazepam (Valium) |
In this Chimera session preview, the GABAA receptor is shown in blue (chains A, B, C, D, E). GABA (ABU) is shown in yellow. Diazepam (DZP) is shown in red. The membrane is indicated in the image for reference. The PDB structure also contains a nanobody (chain G) that was added to the protein for crystallization, however it is not a part of biological molecule and it is hidden in this session. |
|
2b. Metabotropic Receptors
Metabotropic receptors, also called G-protein coupled receptors or GPCRs, activate signal transduction pathways upon neurotransmitter binding. All GPCRs have similar structure composed of a bundle of seven alpha helices that span the membrane. The helices form a cavity on the extracellular side where the neurotransmitters bind.
On the intracellular side, the receptors are coupled to G-proteins. G-proteins consist of alpha (Gα), beta (Gβ), and gamma (Gγ) subunits. When inactive, they have GDP bound to the Gα subunit. Ligand binding induces a conformational change in the receptor that leads to the activation of the G-protein. Upon activation, the GDP is released and GTP binds instead and the complex splits into 2 parts: Gα and Gβγ, which interact with other proteins in the signalling pathways.
There are several classes of Gα subunits depending on which protein they interact with. The stimulatory (Gαs) and inhibitory Gα (Gαi) interact with adenylyl cyclase–an enzyme that is responsible for production of the cyclic AMP (cAMP). Gαs stimulates cAMP production while Gαi inhibits the process. cAMP binds to some ion channels directly allowing ions to enter or exit the cell thus modulating the membrane voltage. cAMP is also used to activate channels indirectly via binding to CAMP dependent protein kinases, which in turn bind to other channels stimulating ion traffic.
Notice that the same neurotransmitter can mediate both excitatory and inhibitory neurotransmission, depending on the receptor it interacts with. For example, there are 5 types of dopamine receptors which are distributed in different areas of the brain: D1, D2, D3, D4, and D5. They modulate different pathways relating to tasks like locomotions, memory, attention, impulse control, sleep, etc. The D1 and D5 receptors activation stimulates production of cAMP while the activation of D2, D3, and D4 inhibit it 1.
Learn more:
Molecule of the Month articles:
Adrenergic Receptors
Opioid Receptors
Rhodopsin
Serotonin Receptor
G Proteins
Adenylyl Cyclase
Paper model:
Pharmacological Application: Targeting dopamine receptor in treating schizophrenia
Schizophrenia is implicated with abnormal dopamine signaling. A group of antipsychotic medications termed “atypical” or “second generation” target D2 receptors invoking its inhibitory effect on cAMP production. An example of such a drug is risperidone.
PDB Structure ID |
Structure Description |
Visualization resources |
Topic-specific resources |
D2 dopamine receptor in complex with G-protein |
In this Chimera session preview, the dopamine receptor (chain R, blue) is shown complexed with G-protein. A Gα is shown is orange (chain A), Gβ is shown in darker green (chain B), Gγ is shown in lighter green (chain C). An agonist (08Y, red) is shown in the serotonin binding site. The membrane is indicated on the image for reference. The PDB structure also contains an antibody fragment (chain E) that was added to the protein for crystallization, however it is not a part of biological molecule and it is hidden in this session. Note that the Gα subunit is incomplete. To explore a full length Gα, use the PDB structure 6crk. |
1Biochemistry; Dopamine Receptors Psychopharmacology Institute: Mechanism of Action of Risperidone Molecule of the Month on Adenylyl Cyclase |
|
Structure of the D2 dopamine receptor bound to the atypical antipsychotic drug risperidone |
In this Chimera session preview, the dopamine receptor (chain A, blue) is shown with risperidone (8NU, red) bound in the active site. The membrane is indicated on the image for reference. An engineered version of the receptor was used to solve this structure, with lysozyme (white) inserted in the loop between helices 5 and 6; it is not normally a part of the structure. |
||
The structure of a membrane adenylyl cyclase bound to an activated stimulatory G protein |
![]() In this Chimera session preview, the adenylyl cyclase (chain A, blue) is shown complexed with Gαs (chain B, orange). Both Gαs and Gαi can bind to adenylyl cyclase, stimulating or inhibiting the production of cAMP. The D2 receptor interacts with Gαi. Gαs and Gαi use the same binding site, and research suggests that they are structurally similar, however, some molecular dynamics experiments show that they use different mechanisms in interaction with the adenylyl cyclase (learn more). At present there is no PDB structure capturing the interaction of adenylyl cyclase with Gαi.
|
3. Stopping the signal: neurotransmitter removal
The neurotransmitters usually bind to their receptors for a short time. Once the signal is underway, they detach from the receptors and move freely in the synaptic cleft. To stop them from stimulating the receptors again, our nervous system employs special proteins to either transport them back into the pre-synaptic cell or to destroy them.
Pharmacological Application: Targeting SERT transporter in treating depression
Depression is a neuronal disorder associated with decreased activity of serotonin. Drugs called selective serotonin reuptake inhibitors (e.g. paroxetine/Paxil) block the serotonin transporter so the neurotransmitter can be present longer in the intracellular space stimulating the receptor.
PDB Structure ID |
Structure Description |
Visualization resources |
Topic-specific resources |
Serotonin receptor in complex with G-protein |
In this Chimera session preview, the serotonin receptor (chain S) is shown in blue complexed with G-protein. Gα is shown is orange (chain A), Gβ is shown in darker green (chain B), Gγ is shown in lighter green (chain G). An agonist (yellow) is bound in the serotonin binding site. The membrane is indicated on the image for reference. Note that the Gα subunit is incomplete. To explore a full length Gα, use the PDB structure 6crk. |
Molecule of the Month article on Serotonin Receptor |
|
Structure of human serotonin transporter in the ligand-bound inward-facing conformation |
In this Chimera session preview, the serotonin transporter is shown in pink (chain A). The transporter is in the inward open confirmation. Serotonin-like ligand (HJM) is shown in the biding site. The membrane is indicated in the image for reference. The PDB structure also contains an antibody fragment (chains B, C) that was added to the protein for crystallization, however it's not a part of biological molecule and it's hidden in this session. |
||
Structure of human serotonin transporter in complex with paroxetine |
In this Chimera session preview, the serotonin transporter is shown in pink (chain A). Paroxetine (8PR) is shown in the biding site. The membrane is indicated in the image for reference. The PDB structure also contains an antibody fragments (chains L, H. V) that were added to the protein for crystallization, however they are not a part of biological molecule and are hidden in this session. |
3b. Destructing neurotransmitter through special enzymes
Some neurotransmitters are destroyed by special enzymes. For example, the excitatory neurotransmitter acetylcholine is disposed of by acetylcholinesterase. This neurotransmitter is synthesized inside the neurons from acetate and choline and interacts with acetylcholine receptors. The cholinergic neurons (neurons using mainly acetylcholine in communication) play critical role in memory, learning, and attention. In the synapses between nerve cells and muscle cells, acetylcholine triggers the process of muscle contraction. After its job is done, acetylcholinesterase breaks it back into acetyl and choline.
Pharmacological Application: Targeting Acetylcholinesterase in treating Alzheimer's disease
As we age, the number of cholinergic neurons decrease. This process is linked with the onset of Alzheimer's disease, a disorder manifesting itself as progressive loss of cognitive and behavioral function. A group of drugs known as reversible AChE inhibitors (e.g. galantamine) are used in the treatment of the disease. These drugs inhibit the enzyme temporarily, thereby increasing the levels of acetylcholine that is present in the intraneuronal space.
PDB Structure ID |
Structure Description |
Visualization resources |
Topic-specific resources |
Acetylcholine Receptor |
In this Chimera session preview, the receptor (chains A, B, C, D) is shown in blue. One of acetylcholine binding sites and membrane are indicated on the image for reference. |
Molecule of the Month article on Acetylcholine Receptor |
|
Acetylcholinesterase complexed with acetylcholine |
In this Chimera session preview, the acetylcholinesterase (chains A, B) is shown in green with acetylcholine (ACH, yellow) bound in the active site. The membrane is indicated on the image for reference. |
||
Acetylcholinesterase in complex with galantamine |
In this Chimera session preview, the acetylcholinesterase (chains A, B) is shown in green with the drug galantamine (GNT, red) bound in the active site. The membrane is indicated on the image for reference. |
Comprehensive Resources:
Synaptic transmission: A Four Step Process
Visualization Resources References:
- You can download and open these sessions using UCSF Chimera. Use the tutorials available here and here to edit the sessions, create animations or save pictures.
- Mol* is a web-based molecular viewer. Use the video tutorial Exploring PDB Structures in 3D with Mol*: Introductory Guide to get started with this molecular viewer. Access the full documentation learn about more advanced features. Mol* is accessible from each structure summary page, from the tab “3D View”.