Molecule of the Month: Aspartate Transcarbamoylase
Key biosynthetic enzymes are regulated by their ultimate products through allosteric motions.
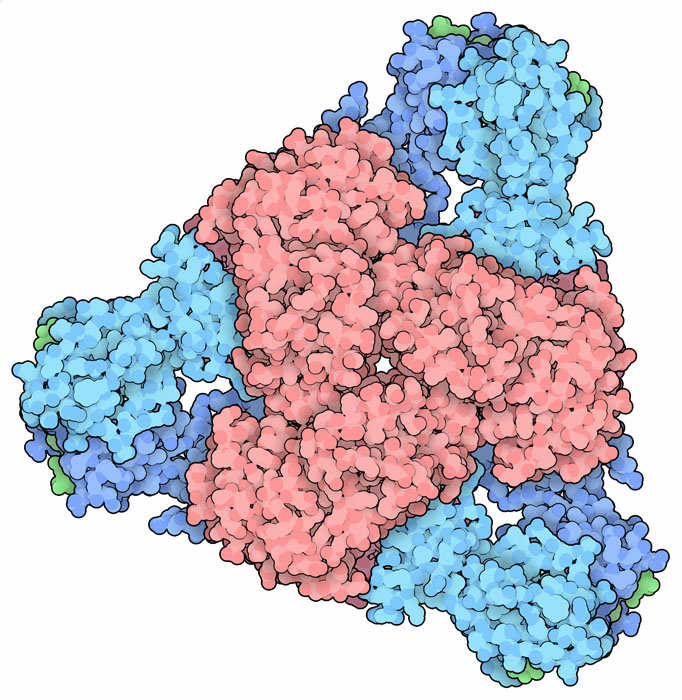
Two States
Choosing the Control Point
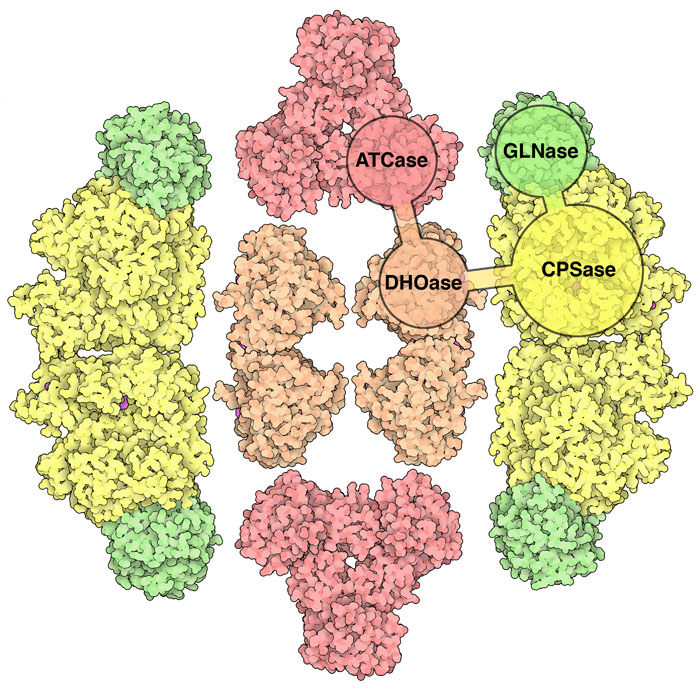
Our Pyrimidine Factory
Exploring the Structure
Aspartate Transcarbamoylase
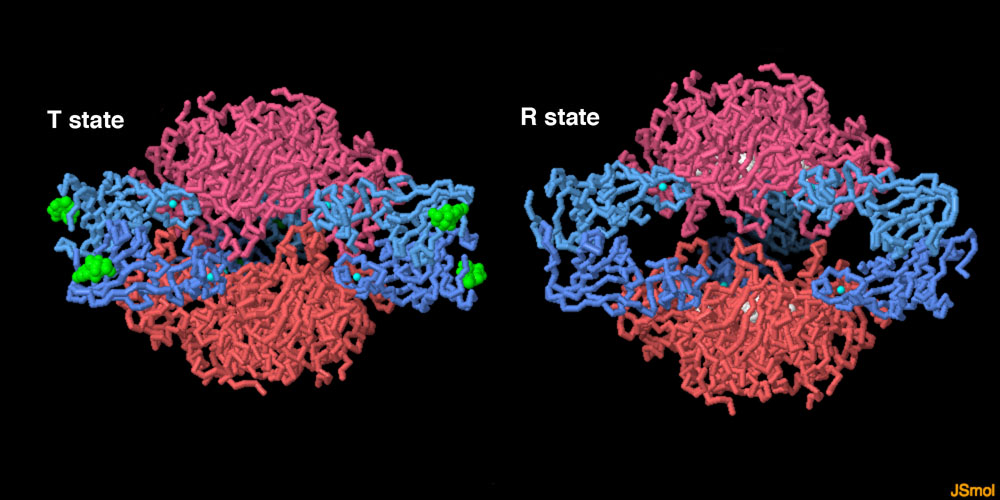
Dozens of structures of ATCase have been determined, capturing the enzyme in both the T and R states, and with a variety of substrates and inhibitors. Two are shown here, showing the amazing allosteric motion that occurs when the enzyme is activated. The T state structure (PDB entry 4fyw) has CTP bound and the R state structure (PDB entry 1d09) has an inhibitor bound in the active site that looks much like the substrates. To explore these two structures in more detail, click on the image for an interactive JSmol. You can also see artistic renderings of the states of ATCase in the Geis Archive.
Topics for Further Discussion
- Many variations on ATCase and the other enzymes of pyrimidine synthesis have been observed in different organisms. For instance, take a look at PDB entry 3d6n for an example of a complex that includes both ATCase and DHOase.
- Many other enzyme pathways are controlled by this type of allosteric regulation. For instance, take a look at phosphofructokinase, which controls breakdown of sugar through glycolysis.
Related PDB-101 Resources
- Browse Enzymes
- Browse Biomolecules
References
- M Moreno-Morcillo, A Grande-Garcia, A Ruiz-Ramos, F del Cano-Ochoa, J. Boskovic & S Ramon-Maiques (2017) Structural insight into the core of CAD, the multifunctional protein leading de novo pyrimidine biosynthesis. Structure 25, 912-923.
- 5g1n: A Ruiz-Ramos, A Velazquez-Campoy, A Grande-Garcia, M Moreno-Morcillo & S Ramon-Maiques (2016) Structure and functional characterization of human aspartate transcarbamylase, the target of the anti-tumoral drug PALA. Structure 24, 1081-1094.
- 5dou: S de Cima, LM Polo, C Diez-Fernandez, AI Martinez, J Cervera, I Fita & V Rubio (2015) Structure of human carbamoyl phosphate synthase: deciphering the on/off switch of human ureagenesis. Scientific Reports 5, 16950.
- 4c6i: A Grande-Garcia, N Lallous, C Diaz-Tejada & S Ramon-Maiques (2014) Structure, functional characterization and evolution of the dihydroorotase domain of human CAD. Structure 22, 185-198.
- 4fyw: GM Cockrell & ER Kantrowitz (2012) Metal ion involvement in the allosteric mechanism of Escherichia coli aspartate transcarbamoylase. Biochemistry 51, 7128-7137.
- WN Lipscomb & ER Kantrowitz (2011) Structure and mechanisms of Escherichia coli aspartate transcarbamylase. Accounts of Chemical Research 45, 444-453.
- 1d09: L Jin, B Stec, WN Lipscomb & ER Kantrowitz (1999) Insights into the mechanisms of catalysis and heterotropic regulation of Escherichia coli aspartate transcarbamoylase based upon a structure of the enzyme complexed with the bisubstrate analogue N-phosphonacetyl-L-aspartate at 2.1 A. Proteins 37, 729-742.
- 5at1: RC Stevens, JE Gouaux & WN Lipscomb (1990) Structural consequences of effector binding to the T state of aspartate carbamoyltransferase: crystal structures of the unligated and ATP- and CTP-complexed enzymes at 2.6-A resolution. Biochemistry 29, 7691-7701.
November 2017, David Goodsell
http://doi.org/10.2210/rcsb_pdb/mom_2017_11