Molecule of the Month: Cisplatin and DNA
Cisplatin treats cancer by causing damage to the DNA of cancer cells.
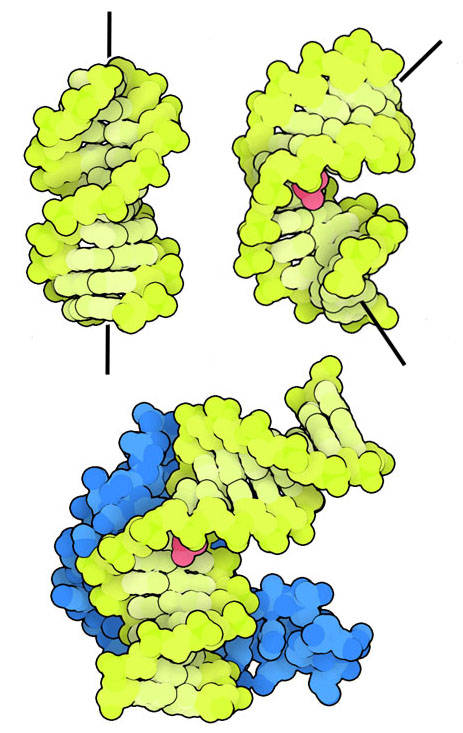
Wonder Drug, Cisplatin
Cisplatin in Action
Cells Resist the Cure?
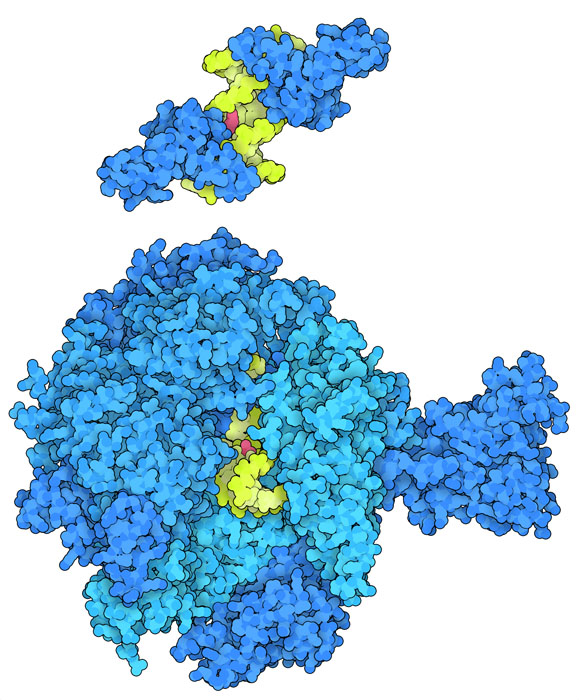
Disrupting Proteins
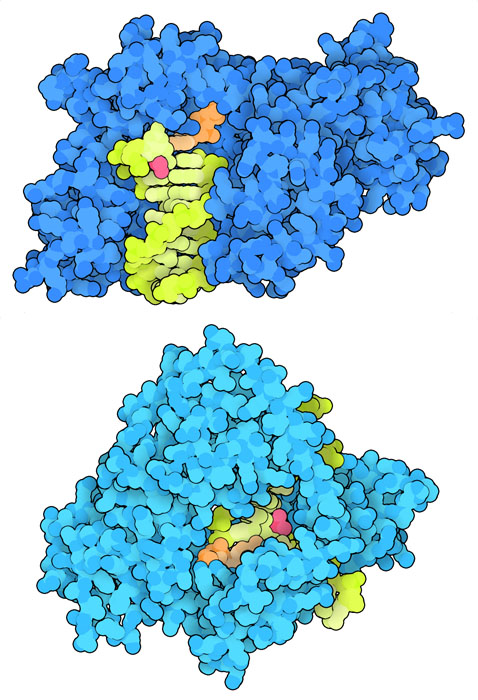
Polymerases: Friend or Foe?
Exploring the Structure
Cisplatin and HMG protein bound to DNA
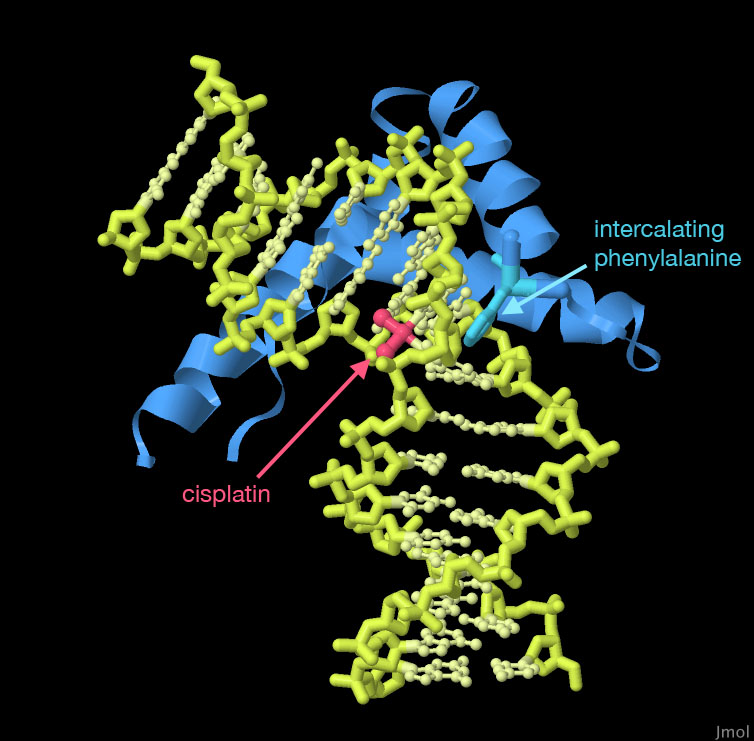
Cisplatin bends DNA by forming covalent bonds between its central platinum and two adjacent guanine bases. PDB entry 1ckt includes a short DNA duplex, a cisplatin lesion and the DNA-binding domain of an HMG protein. HMG proteins also bend the DNA when they bind. Notice how the HMG protein intercalates a phenylalanine (cyan) into the DNA helix at the site of the cisplatin-induced bend. To explore this structure in more detail, click on the image for an interactive JSmol.
Topics for Further Discussion
- Cisplatin triggers rapid degradation of Copper Transporter 1, reducing influx of cisplatin and increasing resistance to it. Explore the transporter in PDB ID 6m97.
- Glutathione transferase (GST) P1-1 is a cisplatin-binding protein that can inactivate cisplatin. Explore it in PDB ID 5djl.
Related PDB-101 Resources
- Browse Cancer
- Browse Central Dogma
References
- 5djl: De Luca, A., Parker, L.J., Ang, W.H., Rodolfo, C., Gabbarini, V., Hancock, N.C., Palone, F., Mazzetti, A.P., Menin, L., Morton, C.J., Parker, M.W., Lo Bello, M., Dyson, P.J. (2019) A structure-based mechanism of cisplatin resistance mediated by Glutathione Transferase P1-1. Proc Natl Acad Sci U S A 116, 13943-13951
- 6m97: Ren, F., Logeman, B.L., Zhang, X., Liu, Y., Thiele, D.J., Yuan, P. (2019) X-ray structures of the high-affinity copper transporter Ctr1. Nat Commun 10: 1386-1386
- 6bs1: Jha, V., Ling, V. (2018) Structural basis for human DNA polymerase kappa to bypass cisplatin intrastrand cross-link (Pt-GG) lesion as an efficient and accurate extender, J Mol Biol 430: 1577-1589
- 5a39: Koch, S.C., Kuper, J., Gasteiger, K.L., Simon, N., Strasser, R., Eisen, D., Geiger, S., Schneider, S., Kisker, C., Carell, T. (2015) Structural insights into the recognition of cisplatin and Aaf-Dg lesion by Rad14 (Xpa). Proc Natl Acad Sci U S A 112: 8272-8277
- Dasari S., Tchounwou P.B. (2014) Cisplatin in cancer therapy: molecular mechanisms of action. Eur J Pharmacol. 740: 364-378
- Shen, D. W., Pouliot, L. M., Hall, M. D., Gottesman, M. M. (2012). Cisplatin resistance: a cellular self-defense mechanism resulting from multiple epigenetic and genetic changes. Pharmacol Rev 64: 706–721
- 3lpv: Todd, R.C., Lippard, S.J. (2010) Structure of duplex DNA containing the cisplatin 1,2-{Pt(NH(3))(2)}(2+)-d(GpG) cross-link at 1.77A resolution. J Inorg Biochem 104: 902-908
- 2r8k: Alt A., Lammens K., Chiocchini C., Lammens A., Pieck J.C., Kuch D., Hopfner K.P., Carell T. (2007) Bypass of DNA lesions generated during anticancer treatment with cisplatin by DNA polymerase eta. Science. 318: 967-970
- 2r7z: Damsma, G.E., Alt, A., Brueckner, F., Carell, T., Cramer, P. (2007) Mechanism of transcriptional stalling at cisplatin-damaged DNA. Nat Struct Mol Biol 14, 1127–1133
- Cohen, S.M., Lippard, S.J. (2001). Cisplatin: from DNA damage to cancer chemotherapy. Prog Nucl Acid Res Mol Biol 67, 93-130
- Kelland, L. (2007) The resurgence of platinum-based cancer chemotherapy. Nat Rev Cancer 7: 573–584
- 1ckt: Ohndorf, U.-M., Rould, M.A., Pabo, C.O., Lippard, S.J. (1999) Basis for recognition of cisplatin-modified DNA by high-mobility-group proteins. Nature 399: 708–712
- Takahara P.M., Rosenzweig A.C., Frederick C.A., Lippard S.J. (1995) Crystal structure of double-stranded DNA containing the major adduct of the anticancer drug cisplatin. Nature 377: 649-52
- 3dnb: Prive, G.G., Yanagi, K., Dickerson, R.E. (1991) Structure of the B-DNA decamer C-C-A-A-C-G-T-T-G-G and comparison with isomorphous decamers C-C-A-A-G-A-T-T-G-G and C-C-A-G-G-C-C-T-G-G. J Mol Biol 217: 177-199
March 2021, Helen Gao, Samuel G. Shrem, Saai Suryanarayanan, David Goodsell
http://doi.org/10.2210/rcsb_pdb/mom_2021_3