Molecule of the Month: SARS-CoV-2 Spike Variants
SARS-CoV-2 is constantly changing, posing new challenges during the COVID19 pandemic
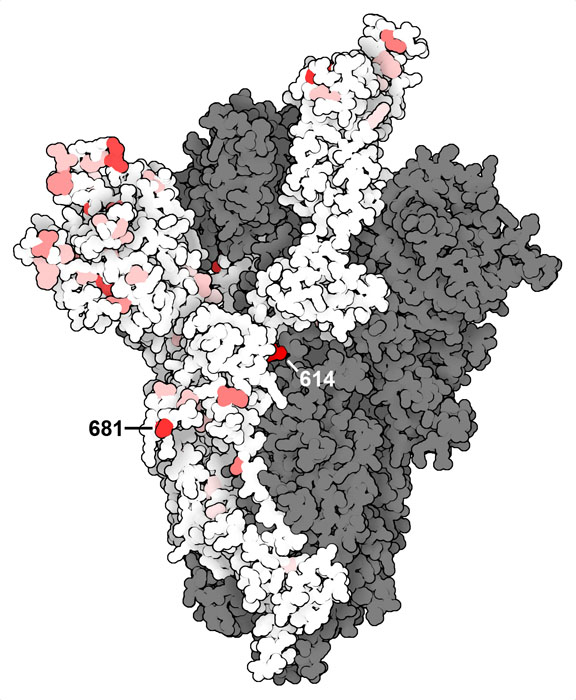
Assessing Variation
Functional Improvements
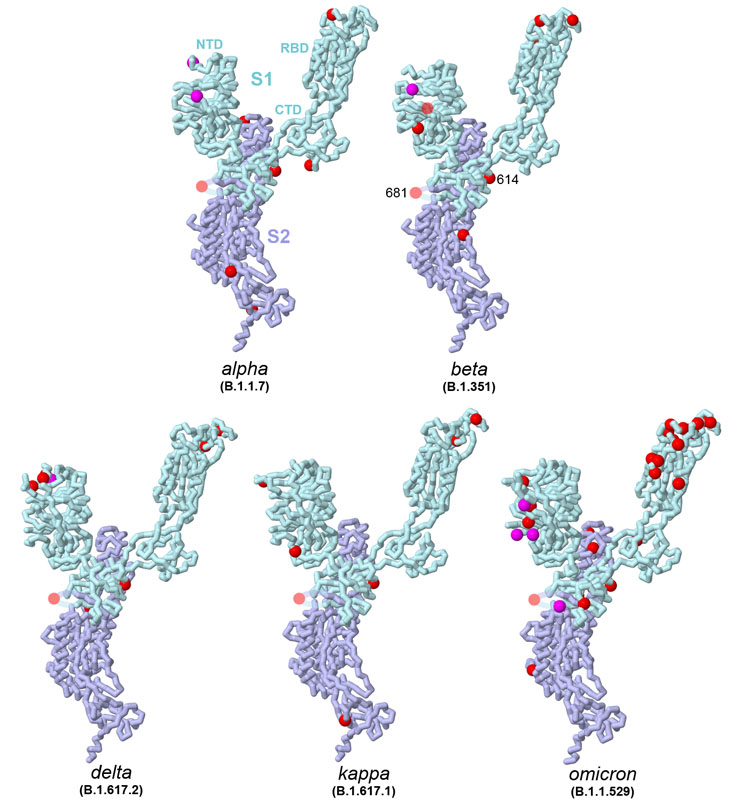
Variant Structures
Exploring the Structure
Spike Variation at Position 614
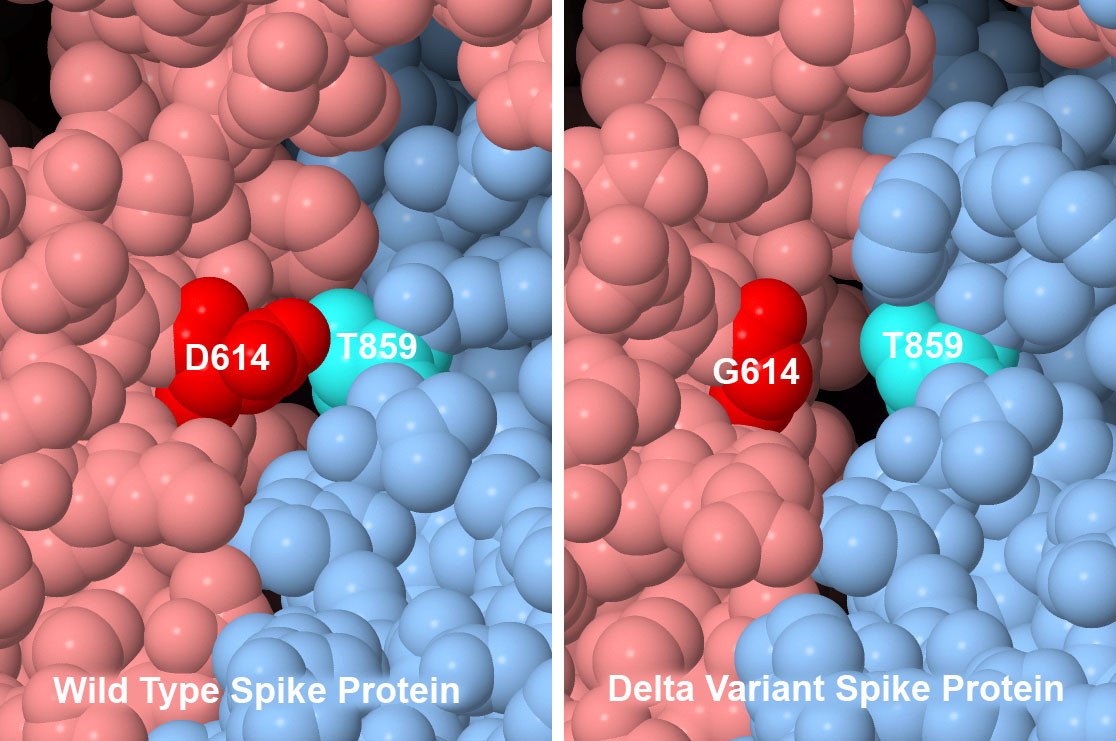
The mutation of aspartate to glycine at position 614 (shown in red) removes an interaction with threonine 859 (turquoise) on a neighboring subunit in the trimeric spike. This is thought to loosen up the structure, making it easier to transition into the active conformation with extended receptor-binding domains. To compare the native structure with aspartate at position 614 (PDB ID 6vyb) and the mutated delta variant structure with glycine (PDB ID 7v7q), click on the image for an interactive JSmol.
Topics for Further Discussion
- Hundreds of structures of SARS-CoV-2 spike protein are available in the archive. An easy way to generate a full list is to go to the structure summary page of one example, such as PDB ID 6vxx, and then click on “Find proteins for P0DTC2” in the “Macromolecules/UniProt" section.
- See “COVID-19/SARS-CoV-2 Resources” for a listing of useful materials for exploring the virus and pandemic.
Related PDB-101 Resources
- Browse Coronavirus
References
- 7lwt, 7lyo: Gobeil, S.M., et al. (2021) Effect of natural mutations of SARS-CoV-2 on spike structure, conformation, and antigenicity. Biorxiv DOI: 10.1101/2021.03.11.435037
- Harvey, W.T., et al. (2021) SARS-CoV-2 variants, spike mutations and immune escape. Nat Rev Microbiol 19, 409-424
- Johnson, B.A., et al. (2021) Loss of furin cleavage sites attenuates SARS-CoV-2 pathogenesis. Nat 591, 293-299
- Lubin, J.H., et al. (2021) Evolution of the SARS-CoV-2 proteome in three dimension (3D) during the first 6 months of the COVID-19 pandemic. Prot Struc Func Genet doi: 10.1002/prot.26250
- Sender, R., et al. (2021) The total number and mass of SARS-CoV-2 virions. Proc Natl Acad Sci USA 118, e2024815118
- 7kj2: Xiao, T., et al. (2021) A trimeric human angiotensin-converting enzyme 2 as an anti-SARS-CoV-2 agent. Nat Struct Mol Biol 28: 202-209
- 6vyb: Walls, A.C., et al. (2020) Structure, Function, and Antigenicity of the SARS-CoV-2 Spike Glycoprotein. Cell 181: 281
December 2021, David Goodsell
http://doi.org/10.2210/rcsb_pdb/mom_2021_12