Molecule of the Month: ATM and ATR Kinases
Dividing cells use ATM and ATR kinases to respond to DNA damage.
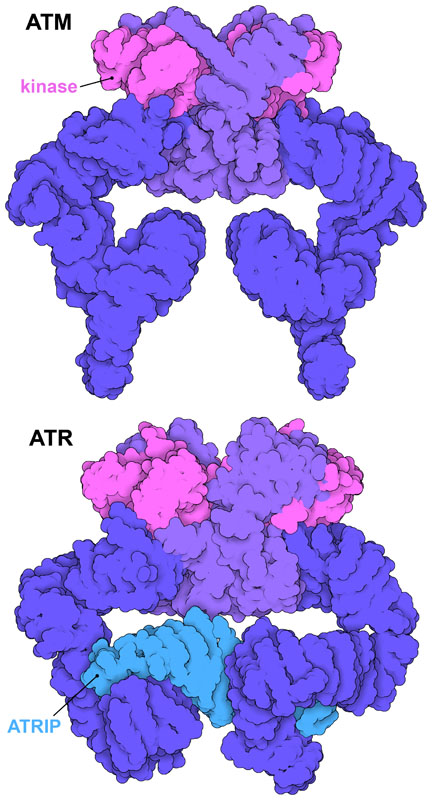
Signaling for Help
Called to Action
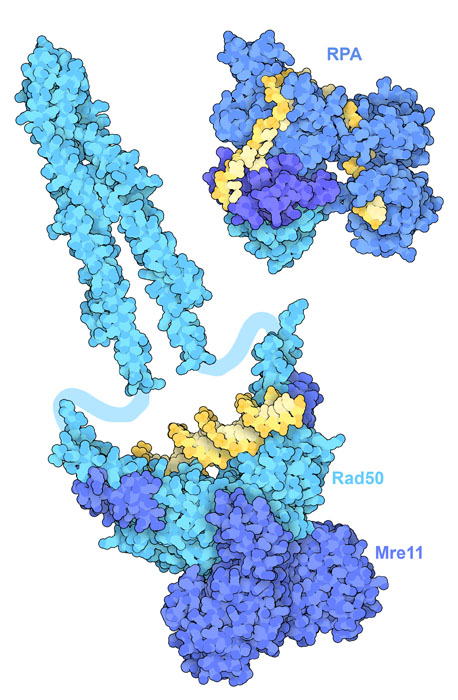
Heroes of the DNA Damage Response
Exploring the Structure
ATM Complexes
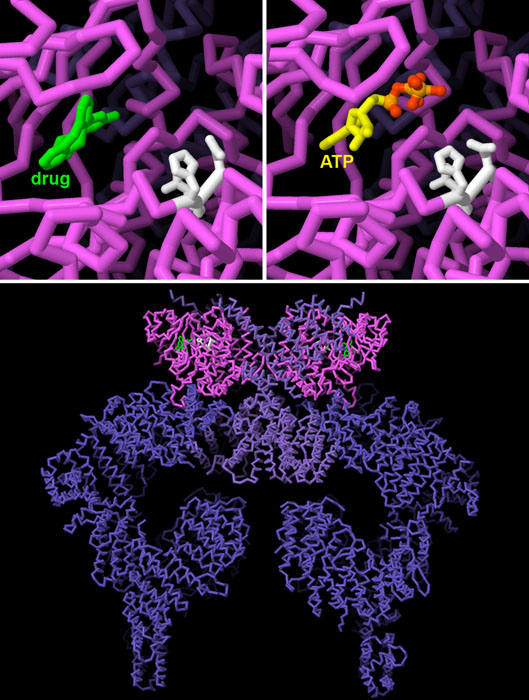
Given their central roles in DNA repair, it is not surprising that ATM and ATR are intimately involved in development of cancer. Cancer cells often have mutated ATM and ATR that fail to stop cell division in cells with damaged genomes. Researchers are taking advantage of this last symptom to design drugs to block ATM. They are now looking for inhibitors to block ATM for use in cancer therapy. Although this may sound paradoxical, these drugs may be injected into tumors to make them more susceptible to radiation therapies. PDB ID 7ni5 includes one of these experimental drugs bound to the kinase domain of ATM. The drug (green) binds in the same place as ATP (yellow, from PDB ID 7ni6) would bind, near three amino acids that catalyze the phosphorylation reaction (white). In the presence of the inhibitor, the enzyme does not bind ATP and cannot perform its kinase activity.
To explore these structures in more detail, click on the image for an interactive JSmol view.
Topics for Further Discussion
- ATM and ATR have been given different names in different organisms. For example, take a look at the yeast protein Tel1 (PDB ID 6s8f) and Mec1-Ddc2 (PDB ID 6z3a).
- You can use the sequence browser to explore the domain structure of these large proteins, which is available in a tab at the top of each Structure Summary Page. For example, here’s the page for ATM.
Related PDB-101 Resources
- Browse Cancer
- Browse Nucleic Acids
- Browse Cellular Signaling
References
- Phan, L. M., Rezaeian, A. H. (2021) ATM: Main features, signaling pathways, and its diverse toles in DNA damage response, tumor suppression, and cancer development. Genes 12, 845.
- 7ni5, 7ni6: Stakyte, K., Rotheneder, M., Lammens, K., Bartho, J. D., Gradler, U., Fuchs, T., Pehl, U., Alt, A., van de Logt, E., Hopfner, K. P. (2021) Molecular basis of human ATM kinase inhibition. Nat Struct Mol Biol 28, 789–798.
- 6z3a: Tannous, E.A., Yates, L.A., Zhang, X., Burgers, P. M. (2021) Mechanism of auto-inhibition and activation of Mec1ATR checkpoint kinase. Nat Struct Mol Biol 28, 50–61.
- Williams, R. M., Yates, L. A., Zhang, X. (2020) Structures and regulations of ATM and ATR, master kinases in genome integrity. Curr Opin Struct Bio 61, 98–105.
- Sun, Y., McCorvie, T. J., Yates, L. A., Zhang, X. (2019) Structural basis of homologous recombination. Cell Molec Life Sci 77, 3–18.
- 5np0: Baretic, D., Pollard, H.K., Fisher, D.I., Johnson, C.M., Santhanam, B., Truman, C.M., Kouba, T., Fersht, A.R., Phillips, C., Williams, R.L. (2017) Structures of closed and open conformations of dimeric human ATM. Sci Adv 3: e1700933-e1700933.
- 5gox: Park, Y.B., Hohl, M., Padjasek, M., Jeong, E., Jin, K.S., Krezel, A., Petrini, J.H.J., Cho, Y. (2017) Eukaryotic Rad50 functions as a rod-shaped dimer. Nat Struct Mol Biol 24: 248-257.
- Rao, Q., Liu, M., Tian, Y., Wu, Z., Hao, Y., Song, L., Qin, Z., Ding, C., Wang, H.-W., Wang, J., Xu, Y. (2017) Cryo-EM structure of human ATR-ATRIP complex. Cell Research 28, 143–156.
- 5dny: Liu, Y., Sung, S., Kim, Y., Li, F., Gwon, G., Jo, A., Kim, A.K., Kim, T., Song, O.K., Lee, S.E., Cho, Y. (2016) ATP-dependent DNA binding, unwinding, and resection by the Mre11/Rad50 complex. EMBO J 35: 743-758.
- Weber, A. M., Ryan, A. J. (2015) ATM and ATR as therapeutic targets in cancer. Pharmacology & therapeutics, 149, 124–138.
- Maréchal, A., Zou, L. (2013) DNA damage sensing by the ATM and ATR kinases. Cold Spring Harbor Persp Biol 5, a012716.
August 2023, Kamiel Beckley, Sarah McGuinness, Asya Polat, Kayla Puebla, David S. Goodsell, Shuchismita Dutta
http://doi.org/10.2210/rcsb_pdb/mom_2023_8